Internet of Vehicles
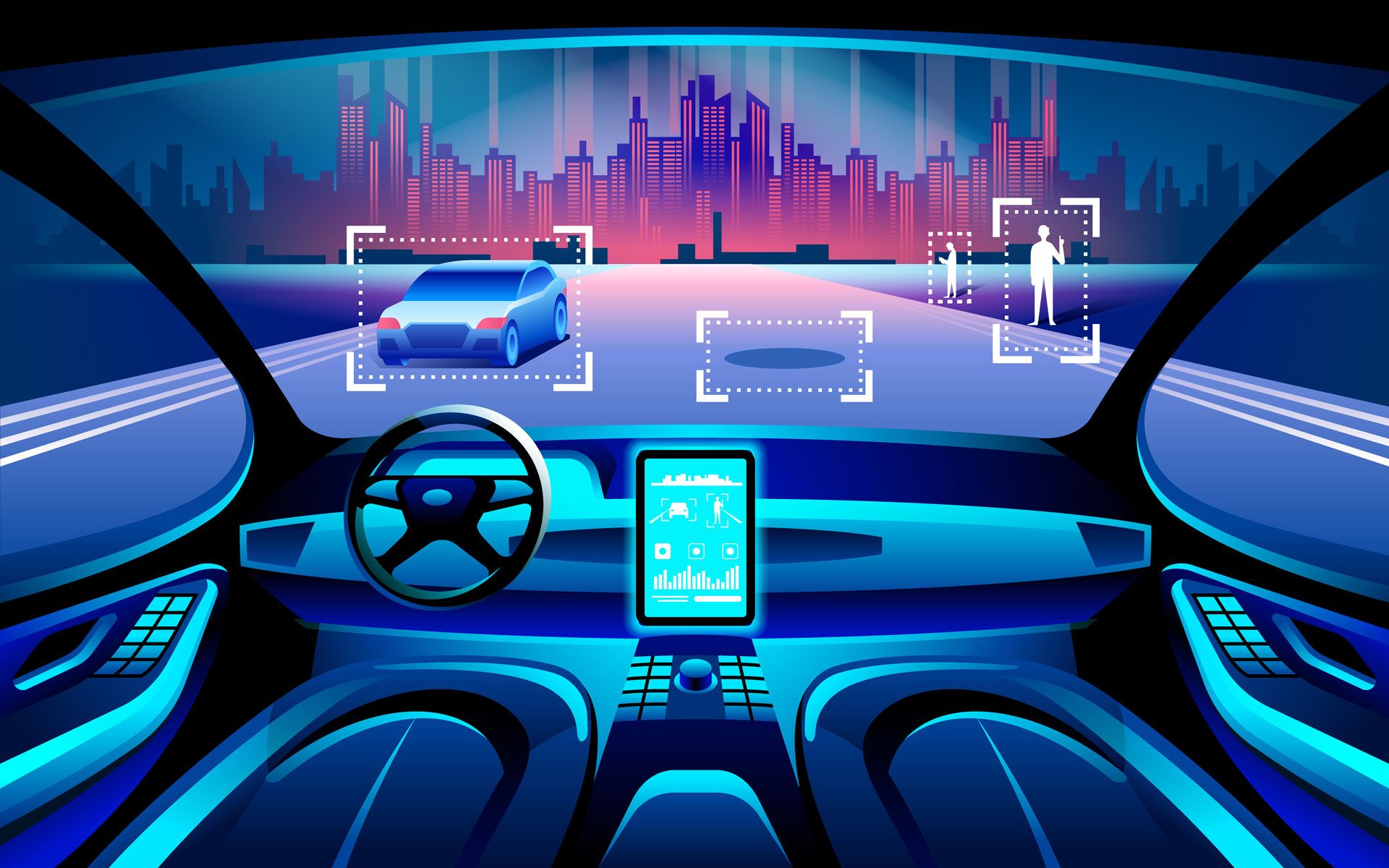
Abstract
This paper will provide an overview of the state of the art of driverless cars, focusing on the communication systems they employ. The technical underpinnings of these technologies will be explored, accompanied by an evaluation. Looking forwards, the future direction for these technologies will be discussed.
Keywords – Autonomous Vehicles, Driverless Cars, Internet of Things (IoT), Internet of Vehicles (IoV), V2x, V2V, V2I, Intelligent Transport System (ITS), Vehicular Ad Hoc Network (VANET).
1. Introduction
Long the stuff of science fiction, driverless cars have captured the public imagination. They offer a convenient solution to unproductive commutes, access to mobility for those that don’t or can’t drive and most promisingly a more efficient and safer way to get around.
Although researchers have been experimenting with autonomous vehicles for almost a century, recent advances in communication technologies culminating in technologies like the Internet of Things (IoT) have propelled the field to new heights by enabling autonomous vehicles to interact with each other and remote hardware. These technologies have been collectively dubbed the Internet of Vehicles (IoV). The integration of communication systems in autonomous vehicles has enabled driverless cars to leverage collective knowledge to perform the driving task more safely and effectively.
The remainder of this paper is split into 4 sections. Section 2 will introduce Vehicular Ad-Hoc Networks and discuss the various technologies underpinning the Internet of Vehicles. In Section 3, seven examples of applications for IoV technology in the context of progressing towards driverless cars will be discussed. Next, Section 4 will provide a Strengths, Weaknesses, Opportunity and Threat (SWOT) evaluation of VANET technology. Finally, Section 5 will make some predictions/recommendations for how the technology can move forwards over the coming decade. As an aside, a brief history of driverless cars is provided in Appendix A.
2. Vehicular Ad-Hoc Networks
Vehicular Ad-Hoc Networks or VANETs are a special case of Mobile Ad-Hoc Networks (MANETS) which support the spontaneous formation of a networks of vehicles. VANETS require three main components [11]:
On-Board Unit (OBU) – This is a device embedded into the vehicle which is capable of communicating with other OBUs and RSUs. It may contain other components such as GPS tracking devices and sensors.
Road-Side Unit (RSU) – These are computing units that are placed along the side of the road and facilitate communication between passing OBUs and wider infrastructure. RSUs can also be used to help vehicles localise themselves and may be powered by an ambient energy source such as solar panels.
Trusted Authority – This is an authority that can be used to validate OBUs and RSUs to ensure that they are honest actors.
VANETS include three main operating modes [11]:
Vehicle-to-Vehicle (V2V) – Also called Inter-Vehicle Communication or IVC, these networks allow vehicles to communicate with each other and share information such as hazards. Various network topologies are available and these networks can be used for Single-hop (SIVC) or Multi-hop (MIVC) configurations. SIVC can be used for short range communications such as lane merging, whilst MIVC are useful for long-range-applications such as traffic condition sharing.
Vehicle-to-Infrastructure (V2I) – Also known as Roadside-to-Vehicle Communication or RVC, these are networks where the vehicle connects to an RSU. RSUs can be used to detect features such as traffic lights or parking meters and data collect from the RSU by the vehicle may be used to manage traffic. Depending on the availability of the infrastructure, these systems can be divided into Sparse RVCs (SRVC) and Ubiquitous RVCs (URVC). SRVCs only provide service at hotspots such as intersections, whilst URVC’s services are always available.
Vehicle-to-Everything (V2x) – These networks allow the vehicle to communicate with other entities such as pedestrians (V2P), devices (V2D) or the grid (V2G). This can be used to help avoid collisions with pedestrians and other road users.
![Figure 1 Vehicle Communication Systems [11]](https://bookstack.jbm.fyi/uploads/images/gallery/2021-11/scaled-1680-/image-1636990112506.png)
2.1 Communication Technologies
There are numerous technologies available that can be used to facilitate VANETs and the IoV. These can be classified by the transmission range of the technology.
2.1.1 Short Range
Short range technologies such as Bluetooth (802.15.1), ZigBee (802.15.4) and Ultra-Wide Band (UWB) do not have sufficient transmission range to support IVC but can be used for Intra-Vehicle Communication such as connecting auxiliary devices like smartphones to the vehicle or eliminating complex wiring from the vehicle. In addition, short range communication technologies could be used to detect close contact between vehicles. [11,12]
2.1.2 Medium Range
2.1.2.1 DSRC
DSRC also known stands for Dedicated Short-Range Communication and is the only wireless communication technology specifically designed for vehicles. DSRC can be used for both V2V and V2I communications and is a modified version of Wi-Fi technology [11]. Numerous territories including the United States [13] and the European Union [14] have designated exclusive radio spectrums for this technology. It has a high reliability and can perform well at high speeds and in poor conditions such as fog, rain and snow. The radio spectrum is divided into one control channel and six service channels with safety critical messages given priority. Security and message privacy are provided by the IEEE 1609.2 standard. However, due to the limited dedicated spectrum, network congestion and broadcast storm can be an issue in busy areas. Due to the relatively limited range of around 300m, only a short-lived connection to RSUs will be possible when moving in SRVC environments and in low vehicle density areas it may not be possible to establish useful V2V VANETS [12]. Biswas et al. was able to show that DSRC is susceptible to Denial-of-Service Attacks [15].
2.1.2.2 Wi-FI
Wi-Fi is a technology that enables Wireless Local Area Networks (WLANs) based on the IEEE 802.11 standards. Wi-fi has a low cost per bit, pervasive global coverage and a high peak throughput, all of which are attractive to vehicles. However, the high mobility of vehicles and low coverage of each individual Wi-Fi access point leads to limited connectivity in vehicles and relegates the technology to a complementary access method to off-load time insensitive payloads. [12]
2.1.3 Long Range
2.1.3.1 Cellular
Cellular networks are now widespread in most developed countries and consist of a number of cells distributed over the land area where each cell is served by a base station (BS). The most widespread cellular network today is the Long-Term Evolution (LTE) system developed by the 3rd Generation Partnership Project (3GPP). Cellular networks can be used to support V2I networks, and since 3GPP release 12, they can also be used for device-to-device networks facilitating usage in V2V networks. 3GPP release 14 added dedicated support for VANETS with C-V2X (Cellular V2X) technology. Cellular technologies can offer reliable connections to vehicles moving at high speeds, and their high capacity makes it possible to support a large number of users in a small geographic area. Vehicles greatly benefit from the high coverage and penetration of cellular technologies and this makes them useful for long lived V2I connections. However, cellular technologies are often expensive to access which leads to a higher cost of transmission then other technologies. In densely populated areas, heavy usage of the cellular network by vehicles may result in a degradation of service quality for other applications. [12]
2.1.3.2 5G-NR
5th Generation New Radio or 5G-NR is the latest standard developed by 3GPP and is an evolution of the existing LTE standard. 5G offers higher data rates, lower latency and enables more devices to connect to the network. This increased capacity helps to address the service degradation mentioned above. 5G offers three new modes that enable additional use cases. Massive Machine Type Communication (mMTC) offers low bandwidth, low energy and high connection density for IoT applications. Enhanced Mobile Broadband (eMBB) offers much higher data rates. Ultra Reliable Low Latency Communications (URLLC) offers extremely low latency and low error rates. All of these modes could be useful for VANETs depending on the specific application. 5G is also interoperable with older 3GPP specifications and so can benefit from existing cellular networks when no 5G coverage is available. [11, 12].
2.2 Heterogeneous VANETs
As seen above, all of the communication technologies available come with a number of limitations and therefore it can often be beneficial to combine them. Heterogeneous VANETs are VANETs that employ at least two different communication technologies. A typical heterogeneous VANET as discussed in [16] would utilise DSRC and cellular technology. In this configuration cellular networks would be used:
As a backup when DSRC MIVC are not available due to sparse vehicle population
A long-range internet access method
A powerful backbone network
[12]
![Figure 2 Heterogeneous VANET [17]](https://bookstack.jbm.fyi/uploads/images/gallery/2021-11/scaled-1680-/image-1636990478687.png)
2.3 Routing Methods
A routing protocol specifies how two entities create and maintain a route to communicate with each other. There are four main categories of VANET routing protocols:
Unicast methods involve a one-to-one transmission between neighbouring entities.
Multicast methods involve a one-to-many or many-to-many transmission between entities.
Broadcast methods involve a one-to-all transmission to all entities in range. These are particularly important in VANETs for safety notifications.
Cluster-based methods use all three of the previously mentioned methods and involve grouping vehicles into clusters. Each cluster has a cluster head (CH) and the rest of the members are cluster members (CM). The CH is responsible for collecting and disseminating information among the cluster, and communicating with other CHs.
[12]
![Figure 3 Routing methods [12]](https://bookstack.jbm.fyi/uploads/images/gallery/2021-11/scaled-1680-/image-1636990585810.png)
3. Applications of Vehicular Networks
The vehicular networks enabled by the communication technologies discussed above support a wide variety of applications. As this is an area of active research, an exhaustive list would be impossible to provide, but some examples of applications will be provided below.
3.1 Collision Prevention
Vehicles participating in VANETs can use short range communication technologies, sensors or predictions based on speed and trajectory to detect when they are in danger of colliding with another vehicle or road user. Once this has been detected, manually controlled vehicles can issue a warning to the driver, whilst (partially) autonomous vehicles can brake automatically. In addition, VANET-enabled vehicles could issue a warning to nearby vehicles when they are under heavy breaking. Finally, if the system detects that a collision is imminent, it could deploy safety measures such as air bags and contacting emergency services. [10]
3.2 Elimination of Traffic Infrastructure
Today’s vehicles rely on a wide range of traffic infrastructure, such as traffic lights and signs to make their operations safe. The use of VANET offers to completely eliminate traffic lights as vehicles could co-ordinate between themselves to avoid collisions at intersections without the use of traffic lights [10]. This would also dramatically increase the traffic throughput at intersections. The information currently provided to drivers by road signs such as speed limit could instead be broadcasted to vehicles using RSUs.
3.3 Traffic Management
VANETs could be used to improve traffic flow, reducing congestion and congestion related accidents. This would also have the benefit of reducing travelling times and increasing fuel efficiency. VANETs could co-ordinate in order to intelligently load balance across road networks, as well as avoid congested areas. Additionally, the data provided by such an application could be used to provide more accurate arrival times and control traffic using adaptive speed limits. In such a system, the vehicles act as sensors by reporting their current speed and location to the network. [10]
3.4 Emergency Services
VANETs provide a number of benefits to emergency services. Firstly, the high-resolution data regarding traffic conditions can be leveraged to plan the most efficient route. Secondly, emergency vehicles can broadcast their presence to nearby vehicles so they can move out of the way. Additionally, emergency vehicles could broadcast an intended route, through busy intersections, for example, vehicles could intelligently organise themselves such that the intended path is clear. Finally, law enforcement vehicles could force their marks to pull over automatically. [10]
3.5 Platooning
The capacity of roads could be greatly improved by a technique known as platooning, whereby groups of vehicles form a tight column and closely follow each other. The “platoon” can then communicate with the goal of acting as a single unit. This enables the platoon to derive aerodynamic gains from the slipstream of the vehicles in front, and enables more efficient usage of the existing road network. [10]
3.6 Traveller Information
VANETs allow the sharing of location specific information by vehicles or RSUs such as fuel/ charging station prices, local attractions and food outlets [10]. In addition, warnings for incidents such as road closures or poor conditions such as snow, ice or fog could be shared by vehicles or authorities. Vehicles could also notify authorities and other road users of road defects such as pot holes.
3.7 Entertainment
VANETs can be used to provide entertainment to passengers. This could come in a variety of forms such as multimedia content downloaded or streamed over the internet, or social communication applications. This could enable passengers to communicate with others in nearby vehicles using V2V connections, or with anyone in the world over the internet.
4. Evaluation
4.1 Strengths
VANETs enable the creation of dynamic and self-organising networks that have the potential to enable a wide variety of applications that can improve safety and quality of life for commuters. They also enable the optimisation of resource utilisation and the leveraging of collective knowledge. Finally, they have the potential to make transport more resilient and intelligent.
4.2 Weaknesses
Many of the useful applications for VANETs will require significant investment in infrastructure, therefore, debate over funding will be likely. Most of the applications of VANETs have privacy implications and commuters may be wary about having their location and other sensitive information shared over the network. The current legal and regulatory environment makes deploying some of these technologies challenging.
4.3 Opportunities
VANETs have the capacity to make the roads safer by avoiding collisions and assisting emergency services. Travel can be made more efficient by increasing road capacities, decreasing congestion and planning better routes. They can make travelling more convenient by improving access to mobility and providing location specific information, as well as more enjoyable by providing entertainment to passengers. VANETs are an important tool in the arsenal towards achieving the goal of fully automated (level 5) vehicles.
4.4 Threats
The automation and connection of vehicles to the internet brings a host of security concerns. Any vulnerabilities in security critical systems could be extremely damaging, resulting in injury or death and these vulnerabilities would be an attractive target for criminals. Legislation, regulation and liability are all also threats for autonomous vehicles and VANETs. Manufacturers will require an attractive legal and regulatory environment in order to invest in autonomous vehicle technology and users will be dissuaded from purchasing autonomous vehicles if they must bear the liability when mistakes are made. A string of high-profile accidents could also turn public opinion against autonomous vehicles, even if they are statistically much safer than manually driven cars.
5. Future
5.1 Short Term
In the short term, the current generation of manually driven cars could be equipped with basic VANET technologies and sensors to enable them to gather data that could be used to train the next generation of autonomous cars.
VANET technologies could be used to warn the drivers of other vehicles that their vehicle has a defect such a blown tail light.
The technology could make cars more secure by notifying owners that their car is being tampered with and possibly providing footage of the offender. Additionally, owners could immobilise stolen cars remotely.
5.2 Medium Term
and manually driven cars will need to co-exist. This brings a host of new challenges, as human drivers do not necessarily act rationally or predictably. Solutions will need to be developed for this so that the roll out of autonomous cars can begin.
A complete network stack similar to the TCP/IP model will need to be developed and standardised for the IoV such that there is a set of services that can be used by all VANET applications and supported by heterogenous vehicles and communication systems.
As VANET technologies are deployed, the amount of data that exists will increase exponentially. This data will be useful for a variety of applications, most notably training machine learning models which is currently a challenge due to the limited data available.
5.3 Long Term
Autonomous cars and VANETs are an enabling technology for smart cities. Smart cities are an aggregation of numerous technologies that together can improve living conditions and quality of life in urban areas. The connection of smart cities to VANETs will enable vehicles to leverage additional data, sensors and devices.
As the amount of data and computation that autonomous vehicles are required to perform increases, they may need to offload some of the computation or data to cloud resources. The current cloud architecture may not be suitable for the needs of VANETs and this will require solutions from the field of fog computing. Fog computing attempts to provide many of the services of cloud computing as a distributed services. [18]
Software Defined Networking (SDN) attempts to provide dynamic programmatic network configuration by decoupling the control and data planes. This could be particularly useful for VANETs as it would enable the dynamic reallocation of network resources to congested areas and provide a more robust network structure. When applied to vehicular networks, this technique is called Software Define Vehicle Networks (SDVN). [19]
References
[1] |
The Milwaukee Sentinel, 08/12/1926: “Phantom Auto will tour city” |
[2] |
Bel Geddes 1940: Magic Motorways |
[3] |
Keshav Bimbraw 2015: “Autonomous Cars: Past, Present and Future” |
[4] |
Reinhold Behringer 1998: “Autonomous Road Vehicle Guidance from Autobahnen to Narrow Curves” |
[5] |
Thorpe, Charles, Martial Herbert, Takeo Kanade, and Steven Shafer 1991: “"Toward autonomous driving: the cmu navlab. i. perception."” |
[6] |
Reanne Boersma, Dennis Mica, Bart van Arem, Frank Rieck 2018: “Driverless electric vehicles at Businesspark Rivium near Rotterdam (the Netherlands): from operation on dedicated track since 2005 to public roads in 2020” |
[7] |
Sebastian Thrun 2010: “Toward Robotic Cars” |
[8] |
Gabe Nelson for Automotive News 2015: “Tesla beams down ‘autopilot’ mode to Model S” |
[9] |
Bernard Marr for Forbes 2018: “Key Milestones Of Waymo - Google's Self-Driving Cars” |
[10] |
Mihail L. Sichitiu, Maria Kihl 2008: “Inter-vehicle Communication Systems: a Survey”. |
[11] |
M. Nadeem Ahangar, Qasim Z. Ahmed 1, Fahd A. Khan, Maryam Hafeez 2021: “A Survey of Autonomous Vehicles: Enabling Communication Technologies and Challenges” |
[12] |
Haixia Peng, Le Liang, Xuemin Shen, Geoffrey Ye Li 2019: “Vehicular Communications: A Network Layer Perspective” |
[13] |
FCC 03-324 Standard |
[14] |
ETSI EN 300 674-2-1 Standard |
[15] |
Subir Biswas, Jelena Mišić, Vojislav Mišić 2012: “DDoS attack on WAVE-enabled VANET through synchronization” |
[16] |
Khadige Abboud, Hassan Aboubakr Omar, Weihua Zhuang 2016: “Interworking of DSRC and Cellular Network Technologies for V2X Communications: A Survey” |
[17] |
Ahmed Al-Saadi, Rossi Setchi, Yulia Hicks 2017: “Semantic Reasoning in Cognitive Networks for Heterogeneous Wireless Mesh Systems” |
[18] |
Pranav Kumar Singh, Sunit Kumar Nandi, Sukumar Nandi 2019: “A tutorial survey on vehicular communication state of the art, and future research directions” |
[19] |
Wafa Ben Jaballah, Mauro Conti, Chhagan Lal 2019: “Software-Defined VANETs:Benefits, Challenges, and Future Directions” |
Appendix A: History
The first experiments with autonomous cars were conducted in the 1920s [1], however these were closer to radio controlled cars than truly autonomous vehicles. The 1930s saw radio-controlled cars powered by electromagnetic fields embedded in the road. This inspired Bel Geddes to argue that humans should be removed from the driving process in his 1940 book [2]. In 1953, RCA Labs built a miniature car guided by wires under the road surface. This was extended to full size in 1958 [3]. The next major leap came in the 1980s when Ernst Dickmann and his colleagues at the University Bundeswehr Munich built a robotic van which navigated using computer vision. It was capable of traveling at up to 63 km/h on roads without traffic [3]. In the same decade EUREKA and the US DARPA agency began researching driverless cars, and DARPA was able to demonstrate a road-following autonomous car using LIDAR and computer vision. The 1990s saw funding pouring into both public and private attempts at driverless cars and saw numerous important achievements. The US department of Transport began research on an automated vehicle and highway system but later dropped this project due to lack of funding. Ernst Dickmann demonstrated two robotic vehicles VaMP and Vita-2 capable of driving on a standard highway with traffic in Paris at speeds of up to 130 km/h, albeit with human interventions [4]. Carnegie Mellon University’s Navlab project culminated in a 5,000 km journey in which 98.2% were autonomous. The car used a neural network to control the steering, but the brakes and throttle were still human-controlled [5]. The 2000s saw the first autonomous public transport systems with the introduction of ParkShuttle in the Netherlands [6]. In 2004, DARPA established the Grand Challenge, an autonomous vehicle race through the desert with a US $1 million prize to any finishers, no cars were able to finish. Five vehicles finished the second Grand Challenge in 2005. The third Grand Challenge was held in an urban environment [7]. By the 2010s, most major automobile manufactures had begun serious work on driverless cars. In 2015 Tesla introduced their “Autopilot” feature which enabled hands-free driving on highways, although the driver was still responsible for the car and would have to step in if a dangerous scenario occurred [8]. In 2017 Google’s Waymo project began testing their fully autonomous vehicle on public roads, without any human in the driving seat [9]. The 2020s saw the first autonomous vehicle regulations with EU regulation 2019/2144 and Japan’s Automate Lane Keeping System (ALKS) regulation.
PDF version: https://portfolio.jbm.fyi/static/IoV.pdf